Mis à jour le 15 Juin 2018
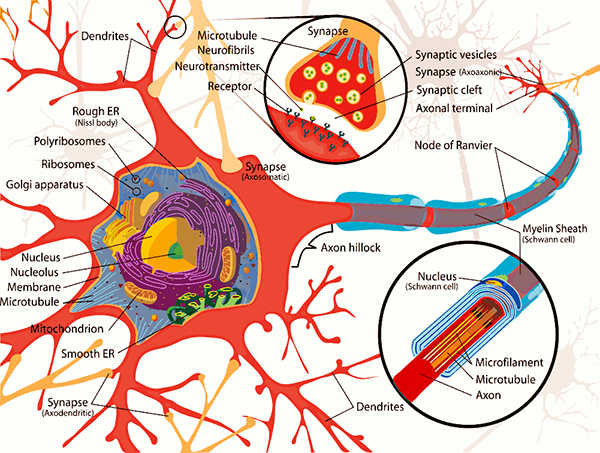
(Image: WPClipArt)
As vast as our universe is, so are its complexities. One of the most complex of objects in it remains the human brain, an organ which when fully grown requires 750 millilitres of oxygenated blood every minute to maintain normal activity – of the total amount of oxygen delivered to the body’s tissues by the arteries, 20 % is consumed by the brain which only makes up 2% of the body’s weight. It also has 100 billion neurons with each connected to 7000 others, leading to a surprising 700 trillion connections. This complexity is far from excessive as we study the importance of the construction of the brain for civilisation and all life on our planet. This fascinating organ is not only at the basis of low-level biological tasks such as heart rate monitoring, respiration and feeding, but it is also vital in the evolution of our behaviours for survival (e.g. perceiving, learning and making rapid decisions). At the heart of human existence, it is also the organ allowing the human organism to explore higher abilities unique to its kind such as thoughts, emotions, consciousness and love.
While nearly 50% of the Central Nervous System and Peripheral Nervous System are neurons, they are supported by glial cells. The ratio of Neurons to glial cells in the human brain is close to 1:1 (Azevedo et al., 2009) and glial cells come in 3 important types:
Firstly, astrocytes [also known as ‘star cell’] produce chemicals needed for neurons to function such as extracellular fluid, provide nourishment [linked to blood vessel] and clean up dead neurons. They also help keep the neuron in place.
Secondly, oligodendrocytes support the axon by creating a myelin coating which increases the speed and efficiency of axonal conduction [in the PNS myelin is produced by Schwann cells].
Thirdly and lastly, microglia works with the immune system by protecting the brain from infections while also being responsible for inflammation in cases of brain damage.
Neurons are cells that are devised to ensure the reception, conduction, and transmission of electrochemical signals and come in several types depending on their structure and function. The 3 main types are Multipolar, Bipolar and Unipolar neurons.
Most neurons in the brain are multipolar, and these have many extensions from their body: one axon and several dendrites. Bipolar neurons have two extensions: one consisting of dendrites and one of axon and are typically specialised sensory pathways (e.g. vision, smell, sight and hearing). Unipolar neurons are cells with a single extension (an axon) from their body and are mostly somatosensory (e.g. touch, pain, temperature, etc). Although existing in variety, all neurons perform the same overall function: to process and transmit information.
The neuron is composed of three main parts, firstly the cell body [also known as the ‘soma’] is a primary component of the neuron that integrates the inputs received by the neurons to the axon hillock. The body or soma is between 5 and 100 microns in diameter (a micron is one-thousandth of a millimetre) surrounded by a membrane and hosts the cytoplasm, the nucleus and a number of organelles. The cytoplasm resembles jelly-like substances and is in continuous movement, with the nucleus containing the genetic code of the neuron that is used for protein synthesis (e.g. of some types of neurotransmitters). The neuron’s metabolism is dependent on the organelles that perform chemical synthesis, generate and store energy; and provide the structural support (similar to a skeleton) for the neuron.
Secondly, we have the dendrites [derived from Greek ‘Dendron’] which are branched cellular extensions emanating from the cell body that receive most of the synaptic contacts from other neurons. It is important to note that dendrites only receive information from other neurons and cannot transmit any of it to them; their purpose is to propagate information to the axon.
Thirdly, axons which can measure from up to a few millimetres to one metre in length, transmit information from the soma to other neurons, ending with the terminal buttons which store chemicals used for inter-neuron communication. There are 2 types of axons. The first type – myelinated axons – are covered with a fatty, white substance known as myelin which is a sheath that has gaps at places known as the nodes of Ranvier. Myelin acts as a catalyst in making electric transmission faster and more efficient by insulating the axon. Hence, with myelinated axons, myelin is vital for effective electric transmission, and its loss leads to serious neurological diseases such as multiple sclerosis, The second type of axons are not covered by myelin, resulting in a slower electric transmission.
Neurons are always active, even when no information is being received from other neurons, and must feed themselves (through blood vessels), maintain physiological parameters within a certain range (homeostasis), and maintain their electrical equilibrium, which is essential in the transmission of information.
The terminal buttons [also known as Axon terminals] are button-like endings of the axon branches which release the information to other neurons via neurotransmitter molecules through synaptic vesicles stored within itself. The neurotransmitter is then diffused across the synaptic cleft [gap between 2 membranes] where a depolarisation from incoming action potentials lead to the opening of Calcium channels and Ca+ triggers vesicles to fuse with pre-synaptic membrane, releasing the neurotransmitter into the synaptic cleft which diffuses across and binds with receptors of the next neuron’s post-synaptic membrane’s receptors; causing particular ion channels to open.
Post synaptic potentials further defines the opening credentials. Excitatory Post Synaptic Potential (EPSP) is the result of depolarisation (+ve) which increases the positive charge after allowing Sodium (Na+) ions inside. Another result could be an Inhibitory Post Synaptic Potential (IPSP) which would be caused by the hyperpolarisation (-ve) due to the opening of Chloride (Cl-) channels. The summation carried out by the Axon Hillock calculates whether it reaches the threshold, if it does; an Action Potential in the Postsynaptic Neuron is triggered and excess neurotransmitter is taken back by the pre-synaptic neuron and degraded by enzymes.
The Neural Signature of Learning
Learning is the process through which memories are formed and it is assumed to be the result of enduring changes in the synapses between neurons – a mechanism called long-term potentiation (LTP), which is the strengthening of connections between two neurons by the synaptic chemical change. Memory storage is the strengthening or weakening of synaptic connections. Hebbian learning is a key principle for long-term potentiation (LTP): “neurons that fire together, wire together” (Hebb, 1949), meaning that any two cells or system of cells that are repeatedly active at the same time will tend to become ‘associated’ – and recent studies seem to also suggest that the growth of new synapses foster learning. A new memory is a change to the nervous system as a result of learning, i.e. a memory is the internal representation of knowledge acquired through experience.
New experiences change the nervous system, a phenomenon known as “neuroplasticity”. One solid example of this process of neuroplasticity is given in the study done by Maguire et al. (2000): where the volume of the hippocampus [an area of the brain essential for learning & memory] of London Taxi Drivers were compared with that of a control group, with the hypothesis that extensive experience with spatial navigation and resulting increase in spatial memory might have led to enduring changes in the brain. Eventually, as predicted the hippocampal volume of the London Taxi Drivers was significantly larger than the normal people in the control group. Furthermore, the hippocampal volume in the taxi drivers correlated positively with the amount of time spent on the job. From such an experiment, it was deduced that new experiences can still change the nervous system in adulthood.
Hebb argued convincingly that enduring changes in the efficiency of synaptic transmission were the basis of long-term memory. If we assume that the repetition of a reverberatory activity induces lasting cellular changes that adds to its stability when an axon of Cell A is near enough to excite a Cell B and repeatedly takes part in its activation, some growth process or metabolic change takes place in one or both cells such that Cell A’s efficiency as one of the cells activating Cell B is increased.
Scientific evidence for Hebb’s law has been repeatedly found, i.e. when a neuron fires, an action potential travels to the end of the axon, where synaptic vesicles release neurotransmitters into the synaptic cleft, these neurotransmitters bind to the postsynaptic receptors on dendrite and trigger an action potential in the next neuron. The strength of such a synaptic connection between neurons is not fixed, but depends on the amount of postsynaptic receptors, the sensitivity of the postsynaptic receptors and the amount of neurotransmitters released by the presynaptic neuron. Correlated activity of presynaptic and postsynaptic neurons result in an increase in the strength of this synaptic connection between neurons, known as long-term potentiation [first observed by Terje Lomo in 1966]. This is the neural signature of learning.
A neuron codes information through its “spiking rate” [response rate] which is the number of action potentials propagated per second. Some neurons may have a high spiking rate in some situations (e.g. during speech), but not others (e.g. during vision), while others may simply have a complementary profile. Neurons that respond to the same type of information are generally grouped together, this leads to the functional specialisation of brain regions. The input a neuron receives and the output that it sends to another neuron is related to the type of information a neuron carries. For example, information about sounds is only processed by the primary auditory cortex because this region’s inputs are from a pathway originating in the cochlea and they also send information to other neurons involved in a more advanced stage of auditory processing (e.g. speech perception). For example, if it were possible to rewire the brain such that the primary auditory cortex was to receive inputs from the retinal pathway instead of the auditory pathway (Sur & Leamey, 2001), the function of that part of the brain would have changed [along with the type of information it carries] even if the regions themselves remained static [with only inputs rewired]. This is worthy of being noted as when one considers the function of a particular cerebral region: the function of any brain region is determined by its inputs and outputs – hence, the extent to which a function can only be achieved at a particular location is a subject open to debate.
Gray matter, white matter and cerebrospinal fluid
Neurons in the brain are structured to form white matter [axons and support cells: glia] and gray matter [neuronal cell bodies]. The white matter lies underneath the highly convoluted folded sheet of gray matter [cerebral cortex]. Beneath the white matter fibers, there is another collection of gray matter structures [subcortex], which includes the basal ganglia, the limbic system, and the diencephalon. White matter tracts may project between different regions of the cortex within the same hemisphere [known as association tracts) and also between regions across different hemispheres [known as commissures; with the most important being the corpus callosum]; or may project between cortical and subcortical regions [known as projection tracts]. A number of hollow chambers called ventricles also form part of the brain, these are filled with cerebrospinal fluid (CSF), which serves important functions such as carrying waste metabolites, transferring messenger signals while providing a protective cushion for the brain.
Reflections: From biology to psychology
In the classic essay on the “Architecture of Complexity”, Simon (1996) noted that hierarchies are present everywhere at every level in natural systems – taking the field of physics as an example, in particular the way elementary particles form atoms, atoms form molecules, and molecules form more complex entities such as rocks. Furthering this metaphor as an example, we may also wish to look at the organisation of a book: letters, words, sentences, paragraphs, sections and finally chapters.
In biological systems, a similar type of hierarchical structure can be found at many levels, particularly in the way the brain is organised. Simon seems to convincingly argue that complex systems’ evolution would have had to have benefited from some degree of stability, which is precisely enabled by hierarchical organisation. The main idea is that hierarchical organisations typically have a degree of redundancy – that is, the same functions at the particular level can be carried out by different components; and if one component fails, the system is only slightly affected since other components could perform the functions to some extent. Systems that lack systematic hierarchical organisation tend to lack this degree of flexibility, and a system as complex as the human brain must have a strong hierarchical organisation, or it would not have been able to evolve into such a complex organ.
Using the Limbic system [diagram above] as an example of each level’s specialisation, it is possible to understand how it is responsible for a particular set of functions related but also separate from other parts of the brain. The Limbic system is essential in allowing the human organism to relate to its environment based on current needs and the present situation with experience gathered. This very intriguing part of the brain may in fact be the source of – what many might call – “Humanity” in man as it is responsible for the detection and subsequent expression of emotional responses. One of its parts, the amygdala is implicated in the detection of fearful or threatening stimuli, while parts of the cingulate gyrusare involved in the detection of emotional and cognitive conflicts. Another part, the hippocampus is of major importance in learning and memory; it lies buried in the temporal lobes of each hemisphere along with the amygdala. Other structures of the Limbic system are only visible from the ventral surface [underside] of the brain; the mamillary bodies are two small round protrusions that have traditionally been implicated in memory (Dusoir et al., 1990), while the olfactory bulbs are located under the surface of the frontal lobes with their connections to the limbic system underscoring the importance of smell for detecting environmentally salient stimuli (e.g. food, animals, cattle, cars, etc) and its influence on mood and memory.
One of the main insight of Simon’s analysis is that scientists should be thankful to nature for the existence of hierarchies, since they make the task of understanding the mechanisms involved easier. It can be achieved by simply focusing on one specific level rather than trying to understand the phenomena in all its complexities – because each level has its own laws and principles. On initial approximation, what happens at lower levels may end up being averaged without taking into account all the details and the happenings at the higher levels, which may unfairly be considered as constant.
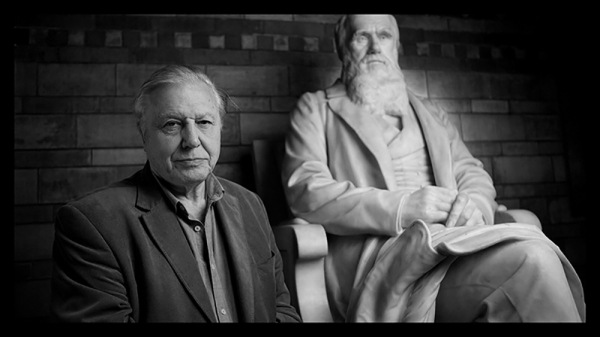
Naturalist, David Attenborough / Image: Darwin & the tree of life (2009)
Focusing on a popular example, we could look at the biologist and naturalist Charles Darwin when he formulated his theory of evolution. At that time, the structure of DNA [which would be discovered 70 years later] was not a major concern of his, furthermore the latter did not have to consider the way the Earth came to exist. Instead, what the biologist did was to focus on an intermediate level in the hierarchy of natural phenomena (e.g. primates, animals, birds, insects, etc): how species evolved over time. Such example also seems to illustrate a vital point in this analysis: the processes involved at the level we are interested in can be understood by analysing the constraints provided by the levels below and above. What happens at the low levels (e.g. the biochemical level) and what happens at high levels (e.g. the cosmological level) limit how any species evolve; and if the biochemistry of life had been disrupted, and if our planet did not provide the appropriate environmental elements and conditions for life to flourish, evolution would simply not have happened. As science progresses and shatters many outdated perspectives at looking at life & nature on planet Earth, links are being made between these different levels of explanation.
It is now firmly accepted among intellectuals from evidence gathered in Biopsychology (also known as Neuroscience) that the acquisition of skills is dependent on an organism’s ability to learn and develop throughout its lifetime, and DNA is an important factor at the biochemical level for the transmission of heredity traits postulated by Charles Darwin. Hence, human evolution is a process that is continuous, multifaceted, complex, creative & ongoing; and intelligent design [e.g. psychological, educational, linguistic, biological, genetic, philosophical, environmental, dietary, etc] is an undeniably important factor for the intelligent evolution of human societies.
****
References
- Azevedo, F.A.C., Carvalho, L.R.B., Grinberg, L.T., Farfel, J.M., Ferretti, R.E.L., Leite, R.E.P., Jacob Filho, W., Lent, R. & Herculano-Houzel, S. (2009) Equal numbers of neuronal and nonneuronal cells make the human brain an isometrically scaled-up primate brain. Journal of Comparative Neurolology , 513 , 532-541.
- Dusoir, H., Kapur, N., Byrnes, D. P., McKinstry, S., & Hoare, R. D. (1990). The role of diencephalic pathology in human-memory disorder-evidence from a penetrating paranasal brain injury. Brain , 113 , 1695-1706.
- Gobet, F., Chassy, P. and Bilalic, M. (2011). Foundations of cognitive psychology. 1st ed. New York: McGraw-Hill Higher Education.
- Hebb, D. O. (1949). Organization of behaviour. NJ: Wiley and Sons.
- Lomo, T. (2003). The discovery of long-term potentiation. Philosophical Transactions of the Royal Society B: Biological Sciences, 358(1432), pp.617-620.
- Maguire, E., Gadian, D., Johnsrude, I., Good, C., Ashburner, J., Frackowiak, R. and Frith, C. (2000). Navigation-related structural change in the hippocampi of taxi drivers. Proceedings of the National Academy of Sciences, 97(8), pp.4398-4403.
- Pinel, J. (2014). Biopsychology 8th ed. Harlow: Pearson.
- Simon, H. A. (1996). The sciences of the artificial (3rd edn). Cambridge: The MIT Press.
- Sur, M. & Leamey, C. A. (2001). Development and plasticity of cortical areas and networks. Nature Reviews Neuroscience , 2 , 251-262.
Danny J. D’Purb | DPURB.com
____________________________________________________
While the aim of the community at dpurb.com has been & will always be to focus on a modern & progressive culture, human progress, scientific research, philosophical advancement & a future in harmony with our natural environment; the tireless efforts in researching & providing our valued audience the latest & finest information in various fields unfortunately takes its toll on our very human admins, who along with the time sacrificed & the pleasure of contributing in advancing our world through sensitive discussions & progressive ideas, have to deal with the stresses that test even the toughest of minds. Your valued support would ensure our work remains at its standards and remind our admins that their efforts are appreciated while also allowing you to take pride in our journey towards an enlightened human civilization. Your support would benefit a cause that focuses on mankind, current & future generations.
Thank you once again for your time.
Please feel free to support us by considering a donation.
Sincerely,
The Team @ dpurb.com
P.S.
– If you are a group/organization or individual looking for consultancy services, email: info[AT]dpurb.com
– If you need to reach Danny J. D’Purb directly for any other queries or questions, email: danny[AT]dpurb.com [Inbox checked periodically / Responses may take up to 20 days or more depending on his schedule]
Stay connected by linking up with us on Facebook and Twitter
Silence Is Golden: Transient Neural Deactivation in the Prefrontal Cortex during Attentive Reading
Abstract:
It is becoming increasingly clear that attention-demanding tasks engage not only activation of specific cortical regions but also deactivation of other regions that could interfere with the task at hand. At the same time, electrophysiological studies in animals and humans have found that the participation of cortical regions to cognitive processes translates into local synchronization of rhythmic neural activity at frequencies above 40 Hz (so-called gamma-band synchronization). Such synchronization is seen as a potential facilitator of neural communication and synaptic plasticity. We found evidence that cognitive processes can also involve the disruption of gamma-band activity in high-order brain regions. Intracerebral electroencephalograms were recorded in 3 epileptic patients during 2 reading tasks. Visual presentation of words induced a strong deactivation in a broad (20–150 Hz) frequency range in the left ventral lateral prefrontal cortex, in parallel with gamma-band activations within the reading network, including Broca’s area. The observed energy decrease in neural signals was reproducible across patients. It peaked around 500 ms after stimulus onset and appeared subject to attention-modulated amplification. Our results suggest that cognition might be mediated by a coordinated interaction between regional gamma-band synchronizations and desynchronizations, possibly reflecting enhanced versus reduced local neural communication.
Lachaux, J., Jung, J., Mainy, N., Dreher, J., Bertrand, O., Baciu, M., Minotti, L., Hoffmann, D. and Kahane, P. (2007). Silence Is Golden: Transient Neural Deactivation in the Prefrontal Cortex during Attentive Reading. Cerebral Cortex, 18(2), pp.443-450.
______________________________________________________________
Watching the Brain during Meaning Acquisition
Abstract:
Acquiring the meaning of a new word in a foreign language can be achieved either by rote memorizing or, similar to meaning acquisition during infancy, by extracting it from context. Little is known about the brain mechanisms involved in word learning. Here we demonstrate, using event-related brain potentials, the rapid development of a brain signature related to lexical and semantic processing during contextual word learning. Healthy volunteers engaged in a simple word-learning task were required to discover the meaning of a novel word from a context during silent reading. After 3 exposures, brain potentials to novel words in meaningful contexts were indistinguishable from real words, although this acquisition effect was not observed for novel words, for which sentence contexts allowed no meaning derivation. Furthermore, when the learned novel words were presented in isolation, an activation of their corresponding meaning was observed, although this process was slower than for real words.
Mestres-Misse, A., Rodriguez-Fornells, A. and Munte, T. (2006). Watching the Brain during Meaning Acquisition. Cerebral Cortex, 17(8), pp.1858-1866.
______________________________________________________________
On dit “cerveau” ou “cervelle”?
Lien: https://www.francaisfacile.com/forum/lire.php?num=7&msg=62686&titre=Cerveau+ou+cervelle
______________________________________________________________
On neural correlates of individual differences in novel grammar learning: An fMRI study
Abstract:
We examine the role of language analytical ability, one of the components of language aptitude – a specific ability for learning languages – during acquisition of a novel grammar. We investigated whether the neural basis of Artificial Grammar Learning (AGL) differs between populations of highly and moderately skilled learners. Participants performed an AGL task during an fMRI scan and data from task’s test phases were analysed. Highly skilled learners performed better than moderately skilled ones and engaged during the task more neural resources in the right hemisphere, i.e. in the right angular/supramarginal gyrus and superior frontal and middle frontal gyrus and in the posterior cingulate gyrus. Additional analyses investigating the temporal dynamics of brain activity during learning revealed lateralisation differences in the modulation of activity in the parietal and temporal cortex. In particular, the left angular gyrus BOLD activity was coupled with high performance on the AGL task and with a steep learning curve.
Kepinska, O., de Rover, M., Caspers, J. and Schiller, N. (2017). On neural correlates of individual differences in novel grammar learning: An fMRI study. Neuropsychologia, 98, pp.156-168.
______________________________________________________________
Individual differences in the learning potential of human beings
Abstract:
To the best of our knowledge, the genetic foundations that guide human brain development have not changed fundamentally during the past 50,000 years. However, because of their cognitive potential, humans have changed the world tremendously in the past centuries. They have invented technical devices, institutions that regulate cooperation and competition, and symbol systems, such as script and mathematics, that serve as reasoning tools. The exceptional learning ability of humans allows newborns to adapt to the world they are born into; however, there are tremendous individual differences in learning ability among humans that become obvious in school at the latest. Cognitive psychology has developed models of memory and information processing that attempt to explain how humans learn (general perspective), while the variation among individuals (differential perspective) has been the focus of psychometric intelligence research. Although both lines of research have been proceeding independently, they increasingly converge, as both investigate the concepts of working memory and knowledge construction. This review begins with presenting state-of-the-art research on human information processing and its potential in academic learning. Then, a brief overview of the history of psychometric intelligence research is combined with presenting recent work on the role of intelligence in modern societies and on the nature-nurture debate. Finally, promising approaches to integrating the general and differential perspective will be discussed in the conclusion of this review.
Stern, E. (2017). Individual differences in the learning potential of human beings. npj Science of Learning, 2(1).
______________________________________________________________
Brain Networks of Explicit and Implicit Learning
Abstract:
Are explicit versus implicit learning mechanisms reflected in the brain as distinct neural structures, as previous research indicates, or are they distinguished by brain networks that involve overlapping systems with differential connectivity? In this functional MRI study we examined the neural correlates of explicit and implicit learning of artificial grammar sequences. Using effective connectivity analyses we found that brain networks of different connectivity underlie the two types of learning: while both processes involve activation in a set of cortical and subcortical structures, explicit learners engage a network that uses the insula as a key mediator whereas implicit learners evoke a direct frontal-striatal network. Individual differences in working memory also differentially impact the two types of sequence learning.
Yang, J. and Li, P. (2012). Brain Networks of Explicit and Implicit Learning. PLoS ONE, 7(8), p.e42993.
______________________________________________________________
Developmental Psychology: The 3 Major Theories of Childhood Development
In 1984, Nicholas Humphrey described us as “nature’s psychologists’” or homo psychologicus. What he meant was that as intelligent social beings, we tend to use our knowledge of our own thoughts and feelings – “introspection” – as a guide for understanding how others are likely to think, feel and hence, behave. He also argued that we are conscious [i.e. we have self-awareness] precisely because such an attribute is useful in the process of understanding others and having a successful social existence – consciousness is a biological adaptation that enables us to perform introspective psychology. Today, we are confident in the knowledge that the process of understanding others’ thoughts, feelings and behaviour is an ability that develops through childhood and most likely throughout our lives; and according to the greatest child psychologist of all time, Jean Piaget, a crucial phase of this process occurs in middle childhood.
Developmental psychology can be characterised as the field that attempts to understand and explain the changes that happen over time in the thought, behaviour, reasoning and functioning of a person due to biological, individual and environmental influences. Developmental psychologists study children’s development, and the development of human behaviour across the organism’s lifetime from a variety of different perspectives. Hence, if we are studying different areas of development, different theoretical perspectives will be fundamental and may influence the ways psychologists and scholars think about and study development.
Through the systematic collection of knowledge and experiments, we can develop a greater understanding and awareness of ourselves than would otherwise be possible…
Full Article: https://dpurb.com/2018/07/15/developmental-psychology-the-3-major-theories-of-development/
______________________________________________________________
https://twitter.com/ArtHistoryFeed/status/984507387255148544
Essay // Biopsychology | The Temporal Lobes: Vision, Sound & Awareness
Extract:
The temporal lobe consists of all the tissues located underneath the lateral (Sylvian) fissure and anterior to the occipital cortex (FIGURE A). The subcortical temporal lobe structures include the limbic cortex, the amygdala, and the hippocampal formation (FIGURE B). The connections to and from the temporal lobe extend to all areas of the brain. Typical symptoms of temporal-lobe disorder or damage generally include drastic deficits in affect and personality, memory problems, and some form of deficits of language.
Subdivisions of the Temporal Cortex
10 temporal areas were identified by Brodman, however many more have recently been discovered in monkeys, and this finding suggests that humans too may have many more areas to explore. The temporal areas on the lateral surface can be divided into those that are auditory (FIG. A. (B), Brodman areas 41, 42 and 22) and those that make up the Ventral Visual Stream on the lateral temporal lobe (FIG. A. (B), areas 20, 21, 37 & 38). These regions specific to vision are often referred to as the Inferotemporal Cortex or by von Economo’s designation, TE…
Source: https://dpurb.com/2014/08/19/essay-biopsychology-vision-the-ventral-dorsal-stream/
______________________________________________________________
Neural Correlates of Post-Conventional Moral Reasoning: A Voxel-Based Morphometry Study
Abstract:
Going back to Kohlberg, moral development research affirms that people progress through different stages of moral reasoning as cognitive abilities mature. Individuals at a lower level of moral reasoning judge moral issues mainly based on self-interest (personal interests schema) or based on adherence to laws and rules (maintaining norms schema), whereas individuals at the post-conventional level judge moral issues based on deeper principles and shared ideals. However, the extent to which moral development is reflected in structural brain architecture remains unknown. To investigate this question, we used voxel-based morphometry and examined the brain structure in a sample of 67 Master of Business Administration (MBA) students. Subjects completed the Defining Issues Test (DIT-2) which measures moral development in terms of cognitive schema preference. Results demonstrate that subjects at the post-conventional level of moral reasoning were characterized by increased gray matter volume in the ventromedial prefrontal cortex and subgenual anterior cingulate cortex, compared with subjects at a lower level of moral reasoning. Our findings support an important role for both cognitive and emotional processes in moral reasoning and provide first evidence for individual differences in brain structure according to the stages of moral reasoning first proposed by Kohlberg decades ago.
Prehn, K., Korczykowski, M., Rao, H., Fang, Z., Detre, J. and Robertson, D. (2015). Neural Correlates of Post-Conventional Moral Reasoning: A Voxel-Based Morphometry Study. PLOS ONE, 10(6), p.e0122914.
______________________________________________________________
Neural Correlates of Personalized Spiritual Experiences
Abstract:
Across cultures and throughout history, human beings have reported a variety of spiritual experiences and the concomitant perceived sense of union that transcends one’s ordinary sense of self. Nevertheless, little is known about the underlying neural mechanisms of spiritual experiences, particularly when examined across different traditions and practices. By adapting an individualized guided-imagery task, we investigated neural correlates of personally meaningful spiritual experiences as compared with stressful and neutral-relaxing experiences. We observed in the spiritual condition, as compared with the neutral-relaxing condition, reduced activity in the left inferior parietal lobule (IPL), a result that suggests the IPL may contribute importantly to perceptual processing and self-other representations during spiritual experiences. Compared with stress cues, responses to spiritual cues showed reduced activity in the medial thalamus and caudate, regions associated with sensory and emotional processing. Overall, the study introduces a novel method for investigating brain correlates of personally meaningful spiritual experiences and suggests neural mechanisms associated with broadly defined and personally experienced spirituality.
Miller, L., Balodis, I., McClintock, C., Xu, J., Lacadie, C., Sinha, R. and Potenza, M. (2019). Neural Correlates of Personalized Spiritual Experiences. Cerebral Cortex, 29(6), pp.2331-2338.
______________________________________________________________
Activity in the insula & amygdala predicts *not* changing your mind when evaluating evidence against your beliefs
Abstract:
People often discount evidence that contradicts their firmly held beliefs. However, little is known about the neural mechanisms that govern this behavior. We used neuroimaging to investigate the neural systems involved in maintaining belief in the face of counterevidence, presenting 40 liberals with arguments that contradicted their strongly held political and non-political views. Challenges to political beliefs produced increased activity in the default mode network—a set of interconnected structures associated with self-representation and disengagement from the external world. Trials with greater belief resistance showed increased response in the dorsomedial prefrontal cortex and decreased activity in the orbitofrontal cortex. We also found that participants who changed their minds more showed less BOLD signal in the insula and the amygdala when evaluating counterevidence. These results highlight the role of emotion in belief-change resistance and offer insight into the neural systems involved in belief maintenance, motivated reasoning, and related phenomena.
Article: http://www.nature.com/articles/srep39589
Human sounds convey emotions better than words do
Brain uses “older” systems/structures to preferentially process emotion expressed through vocalizations
It takes just one-tenth of a second for our brains to begin to recognize emotions conveyed by vocalizations, according to researchers from McGill. It doesn’t matter whether the non-verbal sounds are growls of anger, the laughter of happiness or cries of sadness. More importantly, the researchers have also discovered that we pay more attention when an emotion (such as happiness, sadness or anger) is expressed through vocalizations than we do when the same emotion is expressed in speech.
The researchers believe that the speed with which the brain ‘tags’ these vocalizations and the preference given to them compared to language, is due to the potentially crucial role that decoding vocal sounds has played in human survival.
“The identification of emotional vocalizations depends on systems in the brain that are older in evolutionary terms,” says Marc Pell, Director of McGill’s School of Communication Sciences and Disorders and the lead author on the study that was recently published in Biological Psychology. ”Understanding emotions expressed in spoken language, on the other hand, involves more recent brain systems that have evolved as human language developed.”
The researchers were interested in finding out whether the brain responded differently when emotions were expressed through vocalizations (sounds such as growls, laughter or sobbing, where no words are used) or through language. They focused on three basic emotions: anger, sadness and happiness and tested 24 participants by playing a random mix of vocalizations and nonsense speech, e.g. He placktered the tozz, spoken with different emotional intent. (The researchers used nonsense phrases in order to avoid any linguistic cues about emotions.) They asked participants to identify which emotions the speakers were trying to convey and used an EEG to record how quickly and in what ways the brain responded as the participants heard the different types of emotional vocal sounds.
They were able to measure:
1. how the brain responds to emotions expressed through vocalizations compared to spoken language with millisecond precision;
2. whether certain emotions are recognized more quickly through vocalizations than others and produce larger brain responses; and
3. whether people who are anxious are particularly sensitive to emotional voices based on the strength of their brain response.
Anger leaves longer traces – especially for those who are anxious
The researchers found that the participants were able to detect vocalizations of happiness (i.e., laughter) more quickly than vocal sounds conveying either anger or sadness. But, interestingly, they found that angry sounds and angry speech both produced ongoing brain activity that lasted longer than either of the other emotions, suggesting that the brain pays special attention to the importance of anger signals.
“Our data suggest that listeners engage in sustained monitoring of angry voices, irrespective of the form they take, to grasp the significance of potentially threatening events,” says Pell.
The researchers also discovered that individuals who are more anxious have a faster and more heightened response to emotional voices in general than people who are less anxious.
“Vocalizations appear to have the advantage of conveying meaning in a more immediate way than speech,” says Pell. “Our findings are consistent with studies of non-human primates which suggest that vocalizations that are specific to a species are treated preferentially by the neural system over other sounds.”
Pell, M., Rothermich, K., Liu, P., Paulmann, S., Sethi, S. and Rigoulot, S. (2015). Preferential decoding of emotion from human non-linguistic vocalizations versus speech prosody. Biological Psychology, 111, pp.14-25.
Changes in Thickness and Surface Area of the Human Cortex and Their Relationship with Intelligence
Changes in cortical thickness over time have been related to intelligence, but whether changes in cortical surface area are related to general cognitive functioning is unknown. We therefore examined the relationship between intelligence quotient (IQ) and changes in cortical thickness and surface over time in 504 healthy subjects. At 10 years of age, more intelligent children have a slightly thinner cortex than children with a lower IQ. This relationship becomes more pronounced with increasing age: with higher IQ, a faster thinning of the cortex is found over time. In the more intelligent young adults, this relationship reverses so that by the age of 42 a thicker cortex is associated with higher intelligence. In contrast, cortical surface is larger in more intelligent children at the age of 10. The cortical surface is still expanding, reaching its maximum area during adolescence. With higher IQ, cortical expansion is completed at a younger age; and once completed, surface area decreases at a higher rate. These findings suggest that intelligence may be more related to the magnitude and timing of changes in brain structure during development than to brain structure per se, and that the cortex is never completed but shows continuing intelligence-dependent development.
Cortex and IQ: Thickness
Individual differences in intellectual ability were reflected in different age-dependencies for cortical development throughout life (Figs 1 and 2). Higher IQ was associated with more pronounced cortical thinning in the left hemisphere in childhood. During adolescence, the association between IQ and left hemisphere cortical thinning weakened until in adulthood (from around age 21) higher IQ became associated with more pronounced cortical thickening in the left hemisphere, reaching significance at age 29. This was the most evident in the left superior (medial, orbito-) frontal cortex*, superior motor area, gyrus rectus*, Rolandic operculum, Heschl’s gyrus, insula*, and (pre)cuneus (P < 0.05; *significant at FDR = 0.0023; Fig. 3 and see Supplementary Fig. 1). Cortical thickness changes in the right hemisphere showed significant positive associations with IQ only after age 47 (significant in the medial orbitofrontal gyrus at FDR = 0.0074). Higher IQ was associated with an earlier onset of cortical thinning in childhood.
Cortex and IQ: Surface Area
Individuals with higher IQ showed less pronounced (or already completed) surface expansion in both hemispheres in childhood and adolescence and more pronounced surface contraction in adulthood, although the association with IQ reached significance only in adulthood (Figs 1 and 2), particularly for the left and right precentral cortices, the left medial frontal cortex, and the right supramarginal, parietal (superior and inferior) cortices, operculum, and cuneus (all significant at P < FDR = 0.0063; Fig. 3 and see Supplementary Fig. 1). Overall, higher IQ was associated with earlier completion of cortical surface expansion during childhood development.
The surface area was larger in individuals with higher IQ, but the effect decreased with age: from about 3 cm2 per IQ point at age 10 to about 0.7 cm2 per IQ point at age 60 (left) or even vanishing (right).
Separate analyses for males and females showed that the negative association between IQ and change in the surface area remained significant in females (P < 0.01 in both hemispheres for all subjects together), but not in males (P = 0.5 and 0.2 for the left and right hemispheres, respectively). The vanishing association in early adolescence appears to be the result of a (small) positive association in males (n.s.), and a negative association in females (significant up to age 13 in both hemispheres
Cortex and IQ: Thickness and Surface Area
The relationship between the rates of change of cortical thickness and surface area altered with age (Fig. 6). While in general the most rapid changes in the left cortex were found in younger subjects, individuals with the highest intelligence appeared to have the most extreme course in phase-space (Δth/Δt, Δarea/Δt): They showed the most pronounced thinning in adolescence, the largest surface contraction in (young) adulthood, followed by attenuated thinning (and even some thickening) of the cortex at age 30 and above.
In conclusion, we found dynamic changes in cortical thickness and surface area in the age range of 9–60 years that varied by IQ. Although the relationships between intelligence and these 2 measures of the cortex were different, they were linked by a common phenomenon: The greater the developmental change, the higher the intelligence.
These findings suggest that intelligence is more related to the magnitude and timing of brain changes during development than to brain structure per se. The presence of cortical changes at all ages covered by this study also suggests that the development of the cortex is never completed, but rather continues to change depending on someone's intelligence.
Schnack, H., van Haren, N., Brouwer, R., Evans, A., Durston, S., Boomsma, D., Kahn, R. and Hulshoff Pol, H. (2014). Changes in Thickness and Surface Area of the Human Cortex and Their Relationship with Intelligence. Cerebral Cortex, 25(6), pp.1608-1617.
__________________________________________
Genetic and Environmental Influences on the Visual Word Form and Fusiform Face Areas
Two areas of the occipitotemporal cortex show a remarkable hemispheric lateralization: written words activate the visual word form area (VWFA) in the left fusiform gyrus and faces activate a symmetrical site in the right hemisphere, the fusiform face area (FFA). While the lateralization of the VWFA fits with the leftward asymmetry of the speech processing network, origin of the rightward asymmetry for faces is still unclear. Using fMRI data from 64 subjects (including 16 monozygotic (MZ) and 13 dizygotic (DZ) twin pairs), we investigated how activations evoked by written words, faces, and spoken language are co-lateralized in the temporal lobe, and whether this organization reflects genetic factors or individual reading expertise.
We found that the lateralization of the left superior temporal activation for spoken language correlates with the lateralization of occipitotemporal activations for both written words and faces. Behavioral reading scores also modulate the responses to words and faces. Estimation of genetic and environmental contributions shows that activations of the VWFA, the occipital face area, and the temporal speech areas are partially under genetic control whereas activation of the FFA is primarily influenced by individual experience. Our results stress the importance of both genetic factors and acquired expertise in the occipitotemporal organization.
The occipitotemporal cortex has been described as a mosaic of functional preferences (Haxby et al. 2001) comprising distinct and partially specialized cortical sectors for the encoding and recognition of various categories of visual stimuli such as faces, objects, houses, or words. The topological organization of this mosaic is remarkably consistent across the population (Ishai et al. 1999; Hasson et al. 2003).
Two of its prominent peaks present a high degree of hemispheric specialization. First, the activation evoked during the visual presentation of orthographic stimuli is strongly left-lateralized at an invariant position in the left fusiform cortex in most right-handers (Puce et al. 1996; Cohen et al. 2000), regardless of the writing system used (Bolger et al. 2005;Nakamura et al. 2005; Baker et al. 2007). Recent fMRI studies revealed functional specialization for word reading in this region, such as mirror invariance or orthographic sensitivity (Dehaene and Cohen 2011; Pegado et al. 2011; Hamamé et al. 2013; Liu et al. 2013).
Cohen and his collaborators proposed to label this region the visual word form area (VWFA) (Cohen et al. 2000) and suggested that it houses a neural code for written words and pseudowords, although reports of activation of this region by non-word stimuli (Xue et al. 2006; Mei et al. 2010; Kherif et al. 2011) raised a debate concerning this proposal (Dehaene and Cohen 2011; Price and Devlin 2011). Second, the fusiform face area (FFA), a region preferentially activated by faces (Puce et al. 1996; Kanwisher et al. 1997; Yovel et al. 2008), is generally described as showing a preferential lateralization to the right hemisphere (McCarthy et al. 1997; Haxby et al. 1999; Dien 2009), which may however vary with the task (Rossion et al. 2000) and experimental procedure (Mercure et al. 2008). The FFA is a part of a larger ventral network responding to faces (O’toole et al. 2005), which includes a left-hemispheric homolog (lFFA) and bilateral posterior sites in the occipitotemporal cortex, usually referred as the occipital face areas (OFA) (Gauthier et al. 2000; Gobbini and Haxby 2007), which may be crucial for face identification (Schiltz et al. 2006).
The lateralization of visual recognition processes to opposite hemispheres for written words and for faces is supported by several brain lesion studies. While a restricted left-hemispheric lesion of the VWFA may produce pure alexia (Epelbaum et al. 2008), brain-damage restricted to the right fusiform cortex may be sufficient to produce prosopagnosia (Bouvier and Engel 2006; Schiltz et al. 2006).
Intriguingly, the VWFA and right FFA are located at nearly symmetrical positions (Kanwisher et al. 1997; Cohen and Dehaene 2004). The left-hemispheric location of the VWFA is thought to be constrained by its functional links with the spoken language network (Cai et al. 2008; Pinel and Dehaene 2009; Yoncheva et al. 2010), which is known to present a leftward asymmetry early in life, both functionally (Dehaene-Lambertz et al. 2002, 2010) and anatomically (Dubois et al. 2008; Kasprian et al. 2011;Habas et al. 2012), possibly under the influence of genetic factors (Sun et al. 2005; Pinel et al. 2012). For faces, however, the origins of the right-hemispheric lateralization of the FFA remain unknown. It might reflect a generic, early and genetically determined asymmetric organization of the visual brain, for instance for processing low visual frequencies (Woodhead et al. 2011). Alternatively, it might arise as a late consequence of developmental constraints appearing during the specialization of the occipitotemporal cortex, particularly during reading acquisition.
In support of the first view, recent studies showed that the strength of the lateralization of the FFA varies with the subjects’ handedness (Willems et al. 2010). This suggests that a generic trait of asymmetry such as handedness, which also correlates with the lateralization of language (Knecht, Deppe, et al. 2000; Szaflarski et al. 2002), may have a broad impact on several aspects of functional hemispheric specialization, all the way down to visual areas. It is not implausible that the genetic factors that contribute to human handedness (McManus 1991; Francks et al. 2007) have broad influences on the cortex and may therefore impact on both language and FFA lateralization. While it did not directly address the issue of hemispheric asymmetry, an fMRI study of twins showed that the distribution of activity within the occipitotemporal cortex was partially inherited for faces and places, but not for pseudoword recognition (Polk et al. 2007).
According to the second view, the opposite lateralization of the VWFA and the FFA might result from a cortical competition process that would appear late in life, during the acquisition of a new expertise for reading (Dehaene et al. 2010; Cantlon et al. 2011; Scherf et al. 2011). Face and letter string identification share a requirement for detailed foveal processing. In the framework of the neuronal recycling hypothesis (Dehaene and Cohen 2007), it is assumed that learning to read reshapes the specialization profile of ventral visual areas, “recycling” part of the circuitry for invariant object and face recognition and reorienting it to process letters and their combinations (Dehaene 2005; Dehaene and Cohen 2007).
Given that spoken language processing is lateralized to the left hemisphere in most right-handers, this recycling process would occur primarily in the left occipitotemporal cortex, thus displacing the fusiform face-sensitive areas toward the right hemisphere. In support of this view, a recent fMRI study comparing literate versus illiterate adults showed that acquisition of reading expertise induces both an increase in activation in response to visual words, and a reduction in activation to faces within the same left occipitotemporal area (Dehaene et al. 2010). A similar result was observed when comparing normal and impaired 9-year-old readers: not only responses to words were less left-lateralized but responses to faces were less right-lateralized in impaired readers (Monzalvo et al. 2012).
These results, as well as the proximity of fusiform activations for faces and words in the left hemisphere (Puce et al. 1996; Hasson et al. 2002), comfort a model where words and faces compete for the same restricted neural territory (Dehaene 2005; Dehaene and Cohen 2007; Plaut and Behrmann 2011). The VWFA localization would therefore be ultimately determined by bottom-up visual constraints (sensitivity to high-spatial frequencies, foveal inputs, and combinations of contours) (Hasson et al. 2002) and by top-down linguistic inputs (Cai et al. 2008; Pinel and Dehaene 2009), both of which are partially genetically determined. According to this view, we would predict an influence of spoken language lateralization on the occipitotemporal responses to both written words and to faces. We would also predict that the VWFA activation and lateralization might be under as tight genetic control as the FFA (contra Polk et al. 2007).
We investigated the inter-relations of the cortical responses to words, faces, and spoken language in the temporal lobe. Our main goal was to evaluate to what extent the VWFA and FFA activations correlate with the activations to spoken language and whether their organization depends on genetic or environmental factors.
Our results showed that the VWFA activation and lateralization correlate with spoken language activation and lateralization in the posterior and middle STS, as well as with reading expertise. More surprisingly perhaps, we found that the FFA was submitted to similar influences. Analysis of correlations within MZ and DZ twin pairs suggested that while the left VWFA activation was found to be under the influence of both genetic and shared environmental factors, the FFA activation did not present any twin correlation, leading us to the tentative conclusion that FFA activation mostly depends on unique environmental experience.
These new findings may shed some light on the constraints that shape the development of these 2 inferotemporal areas.
Our present findings should encourage more detailed studies aimed at elucidating the origins of the occipitotemporal mechanisms of visual word processing and face recognition.
The observed interactions between ventral face areas and perisylvian language-related regions, while far from being fully understood, suggest that language lateralization, surprisingly, may be one of the primary determinants of the organization, not only of the reading system, but also of the face recognition system.
Pinel, P., Lalanne, C., Bourgeron, T., Fauchereau, F., Poupon, C., Artiges, E., Le Bihan, D., Dehaene-Lambertz, G. and Dehaene, S. (2014). Genetic and Environmental Influences on the Visual Word Form and Fusiform Face Areas. Cereb. Cortex, 25(9), pp.2478-2493.
(Pinel et al., 2014)
__________________________________________
What Kids Should Know About Their Own Brains
Research Findings: Two exploratory studies explored young children’s views of brain function and whether these views can be modified through exposure to a brief classroom intervention. In Study 1, children aged 4–13 years reported that the brain is used for “thinking,” although older children were more likely than younger children to also endorse a role for the brain in sensory activities such as seeing, smelling, or tasting.
This replicates prior findings that young children view brain functioning as being limited to a role in intellectual activities. Study 2 showed that this narrow view of brain function could be broadened through a brief classroom intervention with 1st graders that emphasized connections between the brain and body. Compared with a control condition, the intervention significantly increased awareness of the brain’s involvement in sensory experiences, although it had no effect on children’s responses to stories involving the magical transformation of a protagonist’s brain.
Practice or Policy: Basic aspects of brain function can be taught at the elementary level without requiring a great deal of specialized knowledge on the part of teachers. Such instruction could form an important part of early foundational learning about human biology, an area that is currently neglected in early educational curricula.
Marshall, P. and Comalli, C. (2012). Young Children’s Changing Conceptualizations of Brain Function: Implications for Teaching Neuroscience in Early Elementary Settings. Early Education & Development, 23(1), pp.4-23.
__________________________________________
Cultural variation in the gray matter volume of the prefrontal cortex is moderated by the dopamine D4 receptor gene (DRD4)
Abstract:
Recent evidence suggests a systematic cultural difference in the volume/thickness of prefrontal regions of the brain. However, origins of this difference remain unclear. Here, we addressed this gap by adopting a unique genetic approach. People who carry the 7- or 2-repeat (7/2-R) allele of the dopamine D4 receptor gene (DRD4) are more sensitive to environmental influences, including cultural influences. Therefore, if the difference in brain structure is due to cultural influences, it should be moderated by DRD4. We recruited 132 young adults (both European Americans and Asian-born East Asians). Voxel-based morphometry showed that gray matter (GM) volume of the medial prefrontal cortex and the orbitofrontal cortex was significantly greater among European Americans than among East Asians. Moreover, the difference in GM volume was significantly more pronounced among carriers of the 7/2-R allele of DRD4 than among non-carriers. This pattern was robust in an alternative measure assessing cortical thickness. A further exploratory analysis showed that among East Asian carriers, the number of years spent in the U.S. predicted increased GM volume in the orbitofrontal cortex. The present evidence is consistent with a view that culture shapes the brain by mobilizing epigenetic pathways that are gradually established through socialization and enculturation.
Yu, Q., Abe, N., King, A., Yoon, C., Liberzon, I. and Kitayama, S. (2018). Cultural variation in the gray matter volume of the prefrontal cortex is moderated by the dopamine D4 receptor gene (DRD4)Cultural variation in the gray matter volume of the prefrontal cortex is moderated by the dopamine D4 receptor gene (DRD4). Cerebral Cortex, 29(9), pp.3922-3931.
__________________________________________
Dominant men are faster in decision-making situations and exhibit a distinct neural signal for promptness
Abstract:
Social dominance, the main organizing principle of social hierarchies, facilitates priority access to resources by dominant individuals. Throughout taxa, individuals are more likely to become dominant if they act first in social situations and acting fast may provide evolutionary advantage; yet whether fast decision-making is a behavioral predisposition of dominant persons outside of social contexts is not known. Following characterization of participants for social dominance motivation, we found that, indeed, men high in social dominance respond faster–without loss of accuracy–than those low in dominance across a variety of decision-making tasks. Both groups did not differ in a simple reaction task. Then, we selected a decision-making task and applied high-density electroencephalography (EEG) to assess temporal dynamics of brain activation through event related potentials. We found that promptness to respond in the choice task in dominant individuals is related to a strikingly amplified brain signal at approximately 240 ms post-stimulus presentation. Source imaging analyses identified higher activity in the left insula and in the cingulate, right inferior temporal and right angular gyri in high than in low dominance participants. Our findings suggest that promptness to respond in choice situations, regardless of social context, is a biomarker for social disposition.
da Cruz, J., Rodrigues, J., Thoresen, J., Chicherov, V., Figueiredo, P., Herzog, M. and Sandi, C. (2018). Dominant men are faster in decision-making situations and exhibit a distinct neural signal for promptness. Cerebral Cortex, 28(10), pp.3740-3751.
__________________________________________
Learning-Induced Changes in the Cerebral Processing of Voice Identity
Abstract:
Temporal voice areas showing a larger activity for vocal than non-vocal sounds have been identified along the superior temporal sulcus (STS); more voice-sensitive areas have been described in frontal and parietal lobes. Yet, the role of voice-sensitive regions in representing voice identity remains unclear. Using a functional magnetic resonance adaptation design, we aimed at disentangling acoustic- from identity-based representations of voices. Sixteen participants were scanned while listening to pairs of voices drawn from morphed continua between 2 initially unfamiliar voices, before and after a voice learning phase. In a given pair, the first and second stimuli could be identical or acoustically different and, at the second session, perceptually similar or different. At both sessions, right mid-STS/superior temporal gyrus (STG) and superior temporal pole (sTP) showed sensitivity to acoustical changes. Critically, voice learning induced changes in the acoustical processing of voices in inferior frontal cortices (IFCs). At the second session only, right IFC and left cingulate gyrus showed sensitivity to changes in perceived identity. The processing of voice identity appears to be subserved by a large network of brain areas ranging from the sTP, involved in an acoustic-based representation of unfamiliar voices, to areas along the convexity of the IFC for identity-related processing of familiar voices.
Latinus, M., Crabbe, F. and Belin, P. (2011). Learning-Induced Changes in the Cerebral Processing of Voice Identity. Cerebral Cortex, 21(12), pp.2820-2828.
__________________________________________
The “Creative Right Brain” Revisited: Individual Creativity and Associative Priming in the Right Hemisphere Relate to Hemispheric Asymmetries in Reward Brain Function
Abstract:
The idea that creativity resides in the right cerebral hemisphere is persistent in popular science, but has been widely frowned upon by the scientific community due to little empirical support. Yet, creativity is believed to rely on the ability to combine remote concepts into novel and useful ideas, an ability which would depend on associative processing in the right hemisphere. Moreover, associative processing is modulated by dopamine, and asymmetries in dopamine functionality between hemispheres may imbalance the expression of their implemented cognitive functions. Here, by uniting these largely disconnected concepts, we hypothesize that relatively less dopamine function in the right hemisphere boosts creativity by releasing constraining effects of dopamine on remote associations. Indeed, participants with reduced neural responses in the dopaminergic system of the right hemisphere (estimated by functional MRI in a reward task with positive and negative feedback), displayed higher creativity (estimated by convergent and divergent tasks), and increased associative processing in the right hemisphere (estimated by a lateralized lexical decision task). Our findings offer unprecedented empirical support for a crucial and specific contribution of the right hemisphere to creativity. More importantly our study provides a comprehensive view on potential determinants of human creativity, namely dopamine-related activity and associative processing.
Aberg, K., Doell, K. and Schwartz, S. (2016). The “Creative Right Brain” Revisited: Individual Creativity and Associative Priming in the Right Hemisphere Relate to Hemispheric Asymmetries in Reward Brain Function. Cerebral Cortex, 28(10), pp.4946-4959.
__________________________________________
The Interface Between Language and Attention: Prosodic Focus Marking Recruits a General Attention Network in Spoken Language Comprehension
Abstract:
In spoken language, pitch accent can mark certain information as focus, whereby more attentional resources are allocated to the focused information. Using functional magnetic resonance imaging, this study examined whether pitch accent, used for marking focus, recruited general attention networks during sentence comprehension. In a language task, we independently manipulated the prosody and semantic/pragmatic congruence of sentences. We found that semantic/pragmatic processing affected bilateral inferior and middle frontal gyrus. The prosody manipulation showed bilateral involvement of the superior/inferior parietal cortex, superior and middle temporal cortex, as well as inferior, middle, and posterior parts of the frontal cortex. We compared these regions with attention networks localized in an auditory spatial attention task. Both tasks activated bilateral superior/inferior parietal cortex, superior temporal cortex, and left precentral cortex. Furthermore, an interaction between prosody and congruence was observed in bilateral inferior parietal regions: for incongruent sentences, but not for congruent ones, there was a larger activation if the incongruent word carried a pitch accent, than if it did not. The common activations between the language task and the spatial attention task demonstrate that pitch accent activates a domain general attention network, which is sensitive to semantic/pragmatic aspects of language. Therefore, attention and language comprehension are highly interactive.
Kristensen, L., Wang, L., Petersson, K. and Hagoort, P. (2012). The Interface Between Language and Attention: Prosodic Focus Marking Recruits a General Attention Network in Spoken Language Comprehension. Cerebral Cortex, 23(8), pp.1836-1848.
Scientists reproduce evolutionary changes by manipulating embryonic development of mice
A group of researchers from the University of Helsinki and the Universitat Autònoma de Barcelona have been able to experimentally reproduce morphological changes in mice which took millions of years to occur. in nature Through small and gradual modifications in the embryonic development of mice teeth, induced in the laboratory, scientists have obtained teeth which morphologically are very similar to those observed in the fossil registry of rodent species which separated from mice millions of years ago…
Harjunmaa, E., Seidel, K., Häkkinen, T., Renvoisé, E., Corfe, I., Kallonen, A., Zhang, Z., Evans, A., Mikkola, M., Salazar-Ciudad, I., Klein, O. and Jernvall, J. (2014). Replaying evolutionary transitions from the dental fossil record. Nature.
__________________________________________________
Structural Correlates of Semantic and Phonemic Fluency Ability in First and Second Languages
Abstract:
Category and letter fluency tasks are commonly used clinically to investigate the semantic and phonological processes central to speech production, but the neural correlates of these processes are difficult to establish with functional neuroimaging because of the relatively unconstrained nature of the tasks. This study investigated whether differential performance on semantic (category) and phonemic (letter) fluency in neurologically normal participants was reflected in regional gray matter density. The participants were 59 highly proficient speakers of 2 languages. Our findings corroborate the importance of the left inferior temporal cortex in semantic relative to phonemic fluency and show this effect to be the same in a first language (L1) and second language (L2). Additionally, we show that the pre-supplementary motor area (pre-SMA) and head of caudate bilaterally are associated with phonemic more than semantic fluency, and this effect is stronger for L2 than L1 in the caudate nuclei. To further validate these structural results, we reanalyzed previously reported functional data and found that pre-SMA and left caudate activation was higher for phonemic than semantic fluency. On the basis of our findings, we also predict that lesions to the pre-SMA and caudate nuclei may have a greater impact on phonemic than semantic fluency, particularly in L2 speakers.
Grogan, A., Green, D., Ali, N., Crinion, J. and Price, C. (2009). Structural Correlates of Semantic and Phonemic Fluency Ability in First and Second Languages. Cerebral Cortex, 19(11), pp.2690-2698.
Understanding Dyslexia
Quick Facts About the Causes of Dyslexia:
• A brain-based problem in decoding of written language
• Genetic and hereditary—if you have it there is approximately a 50% chance your child will have it
• Not caused by low intelligence
• Not caused by seeing words backward
• Generally believed to NOT be caused by environmental factors during or after pregnancy
• Often occurs with ADHD but is a separate condition
• With the right kind of program, the brain can be rewired to read more efficiently
• Some specific genes have been identified that play a role, but no genetic fix is on the horizon
More on dyslexia here: http://www.dyslexia-reading-well.com/causes-of-dyslexia.html
__________________________________________
Brain Imaging Reveals Neural Roots of Caring
Ashar, Y., Andrews-Hanna, J., Dimidjian, S. and Wager, T. (2017). Empathic Care and Distress: Predictive Brain Markers and Dissociable Brain Systems. Neuron.
________________________________________
https://twitter.com/NietzscheAcadem/status/878699929757253632
https://twitter.com/NietzscheAcadem/status/856610013825167360
La Jeunesse de Napoleon: L’enfant Prodige, Guerrier Académique
(Radio)active Neurogenesis in the Human Hippocampus
Fifteen years ago, the generation of new neurons in adulthood was documented in the human hippocampus, but lingering questions have remained about the extent of this process. In this issue of Cell, Spalding et al. provide elegant evidence for continued neurogenesis into adulthood at rates that suggest it may play a significant role in human behavior.
Kheirbek, M. and Hen, R. (2017). (Radio)active Neurogenesis in the Human Hippocampus.
__________________________________________
Elucidation of the molecular mechanisms involved in remyelination
Researchers in Japan have revealed the molecular mechanism involved in the process of repair to damage of the myelin sheath.
In vertebrates, axons extending from nerve cells are covered by insulating sheets called the myelin sheath, made with the cell membranes of oligodendrocytes, enabling fast electrical signaling through saltatory conduction. Normally, myelin is repaired, even if damaged, but the mechanism that controls remyelination was not well understood. In addition, in demyelinating diseases such as multiple sclerosis, the myelin sheath does not recover from damage and gets worse, finally leading to symptoms such as vision loss, limb numbness, and movement disorders.
The research group of Professor Masaharu Noda and colleagues of the National Institute for Basic Biology, a member institute of the National Institutes of Natural Sciences, performed a detailed examination of the remyelinating process of damaged myelin using disease model mice. Their results show that a growth factor called pleiotrophin is secreted from nerve axons injured by demyelination, and this pleiotrophin inhibits the function of the receptor molecule PTPRZ of oligodendrocyte precursor cells, stimulating cellular differentiation into oligodendrocytes which form the myelin sheath, thereby promoting remyelination…
Kuboyama, K., Fujikawa, A., Suzuki, R. and Noda, M. (2015). Inactivation of Protein Tyrosine Phosphatase Receptor Type Z by Pleiotrophin Promotes Remyelination through Activation of Differentiation of Oligodendrocyte Precursor Cells. Journal of Neuroscience, 35(35), pp.12162-12171.
Pig Brain Atlas to study Human Brain Development
This website contains the three-dimensional MRI-based averaged brain and atlas of the neonatal piglet (Sus scrofa). This project is a collaboration between the Beckman Institute for Advanced Science and Technology and ACES Department of Animal Science at the University of Illinois Urbana-Champaign.
__________________________________________
#Meditators hv #brains that are physically 7 yrs younger, on average, than non-meditators
http://digest.bps.org.uk/2016/04/experienced-meditators-have-brains-that.html
“I believe in evidence. I believe in observation, measurement and reasoning, confirmed by independent observers. I’ll believe anything, no matter how wild and ridiculous, if there is evidence for it. The wilder and more ridiculous something is however, the firmer and more solid the evidence will have to be.” -Isaac Asimov
__________________________________________
Listening to classical music modulates genes that are responsible for brain functions
A Finnish study group has investigated how listening to classical music affected the gene expression profiles of both musically experienced and inexperienced participants. All the participants listened to W.A. Mozart’s violin concert Nr 3, G-major, K.216 that lasts 20 minutes.
Listening to music enhanced the activity of genes involved in dopamine secretion and transport, synaptic function, learning and memory. One of the most up-regulated genes, synuclein-alpha (SNCA) is a known risk gene for Parkinson’s disease that is located in the strongest linkage region of musical aptitude. SNCA is also known to contribute to song learning in songbirds.
“The up-regulation of several genes that are known to be responsible for song learning and singing in songbirds suggest a shared evolutionary background of sound perception between vocalizing birds and humans”, says Dr. Irma Järvelä, the leader of the study.
In contrast, listening to music down-regulated genes that are associated with neurodegeneration, referring to a neuroprotective role of music.
“The effect was only detectable in musically experienced participants, suggesting the importance of familiarity and experience in mediating music-induced effects”, researchers remark.
The findings give new information about the molecular genetic background of music perception and evolution, and may give further insights about the molecular mechanisms underlying music therapy.
Kanduri, C., Raijas, P., Ahvenainen, M., Philips, A., Ukkola-Vuoti, L., Lähdesmäki, H. and Järvelä, I. (2015). The effect of listening to music on human transcriptome. PeerJ, 3, p.e830.
__________________________________________
A New Research in NeuroImage has revealed that people with artistic orientations have structurally different brains.
This affects their perception along with their general neural processes. However, further research into more details of the source and nature has been halted due to the high cost.
Furthermore, the extent, magnitude, capability and application range of these skills seem to differ from person to person [e.g. acting, learning, writing, visual arts, communication, vocalisation, motor skills, neural processes, etc] depending on a multitude of factors such as genetics, environmental factors, IQ, upbringing, personal intelligence, etc…
__________________________________________
Study of healthy adults finds that 2 types of extroverts have more brain matter than most common brains
#Society #Neuroscience #Evolution #Science #Mind
DOI:10.3758/s13415-014-0331-6
http://goo.gl/7J8Rxj
__________________________________________
#CarlJung #Quotes #Society #Mind #Education #Thoughts #Reasoning #Logic #Reason #People #World
__________________________________________
Psychology: The Concept of Self
The concept of the self will be explored in this essay – where it comes from, what it looks like and how it influences thought and behaviour. Since self and identity are cognitive constructs that influence social interaction and perception, and are themselves partially influenced by society, the material of this essay connects to virtually all aspects of psychological science. The self is an enormously popular focus of research (e.g. Leary and Tangney, 2003; Sedikides and Brewer, 2001; Swann and Bosson, 2010). A 1997 review by Ashmore and Jussim reported 31,000 social psychological publications on the self over a two-decade period to the mid-1990s, and there is now even an International Society for Self and Identity and a scholarly journal imaginatively entitled Self and Identity…
Read in full: https://dpurb.com/2016/01/23/essay-psychology-the-concept-of-self/
Cigarette smoking and thinning of the brain’s cortex
It took ~25 years for complete cortical recovery in affected areas for those at the mean pack-years value in this sample. As the cortex thins with normal aging, our data suggest that smoking is associated with diffuse accelerated cortical thinning, a biomarker of cognitive decline in adults. Although partial recovery appears possible, it can be a long process.
Karama, S., Ducharme, S., Corley, J., Chouinard-Decorte, F., Starr, J., Wardlaw, J., Bastin, M. and Deary, I. (2015). Cigarette smoking and thinning of the brain’s cortex. Molecular Psychiatry, 20(6), pp.778-785.
__________________________________
The “must-be-known” neurobiology of alcohol use disorders
The new topic of the month on our Resource Centre is neurobiology of alcohol use disorders. Continuous progress in technology has resulted in imaging techniques which enable us to visualize brain structure and function, measure neurotransmitters and their receptors, and electrical activity and changes in blood flow in the brain. Techniques like CT, MRI, DTI, PET, SPECT and MEG are yielding unprecedented images and show us what happens to and in the brain for instance when it is activated by emotional stimuli or when exposed to drugs of abuse or pharmacological agents.
Advances in neurobiology paralleled by those made in genetics give us a better understanding of alcohol use disorders and the underlying mechanisms, increasing the body of evidence from which to design new drugs, optimize existing treatment options and identify which patients will respond best to which treatment.
Article: http://progressinmind.elsevierresource.com/neurobiology-alcohol-use-disorders
Superior memorizers employ different neural networks for encoding and recall
Superior memorizers often employ the method of loci (MoL) to memorize large amounts of information. The MoL, known since ancient times, relies on a complex process where information to be memorized is bound to landmarks along mental routes in a previously memorized environment. However, functional magnetic resonance imaging data on groups of trained superior memorizer are rare. Based on the memorizing strategy reported by superior memorizers, we developed a scheme of the processes successively employed during memorizing and recalling digits and relate these to brain activation that is specific for the encoding and recall period. In the examined superior memorizers several regions, suggested to be involved in mental navigation and digit-to-word processing, were specifically activated during encoding: bilateral early visual cortex, retrosplenial cortex, left parahippocampus, left visual cortex, and left superior parietal cortex. Although the scheme suggests that some steps during encoding and recall seem to be analog, none of the encoding areas were specifically activated during the recall. Instead, we found strong activation in left anterior superior temporal gyrus, which we relate to recalling the sequential order of the digits, and right motor cortex that may be related to reciting the digits.
Mallow, J., Bernarding, J., Luchtmann, M., Bethmann, A. and Brechmann, A. (2015). Superior memorizers employ different neural networks for encoding and recall. Front. Syst. Neurosci., 9.
___________________________________
Forgetting is an actively controlled process in the brain
Forgetting Is Regulated via Musashi-Mediated Translational Control of the Arp2/3 Complex
Animals receive and respond to environmental challenges throughout their life. This vast amount of information is retained in the nervous system and ensures the behavioral plasticity of the organism. In order to maintain a highly flexible nervous system, not only the generation of memories but also forgetting (memory loss) is essential to adapt to a constantly changing environment (McGaugh, 2000).
Molecular mechanisms that underlie learning and memory formation are extensively studied, and our current knowledge provides a complex picture on the regulation of synaptic plasticity. The activity-dependent Ca2+ influx during long-term potentiation (LTP), for example, activates a multitude of signaling pathways, trafficking and rearrangements of scaffold proteins (Kessels et al., 2009), protein degradation and synthesis, gene expression changes (Carlezon et al., 2005), and subsequent structural modifications of the actin cytoskeleton (Wang et al., 2006). Modulation of the actin dynamics during learning and memory mediates morphological changes of synaptic areas and is also necessary for the formation of new synaptic connections in vertebrates (Bosch and Hayashi, 2012). However, until now, the molecular mechanisms that link LTP- or long-term depression-regulated signaling cascades to the structural changes of the actin cytoskeleton during learning and memory are poorly investigated.
The two classical psychological concepts of forgetting, decay and interference, are usually thought of as two distinct processes (Jonides et al., 2008, Wixted, 2004). The decay model suggests that memory passively disappears over time, whereas the interference model claims that forgetting results from competition with other memory traces (Jonides et al., 2008, Wixted, 2004). Recent studies demonstrated that active regulation of forgetting likely takes place (Berry et al., 2012, Inoue et al., 2013, Shuai et al., 2010) and that retention and loss of memory does not depend solely upon the activity of kinases and phosphatases. Active regulators of forgetting also include the small guanosine-triphosphate-binding protein Rac in Drosophila (Shuai et al., 2010) and a TIR-1/JNK-1 pathway in the sensory neurons in C. elegans (Inoue et al., 2013). These findings suggest that multiple different signaling cascades are regulating the retention and loss of memories.
RNA-binding proteins (RBPs) have recently emerged as essential modulators of mRNA distribution, translation, and degradation during proper synaptic function (Holt and Bullock, 2009). In vertebrates, musashi1 (msi1) and musashi2 (msi2) are two closely related members of the musashi (msi) gene family, which belongs to the RNA-recognition motif (RRM) containing proteins that interact with single-stranded RNAs (Sakakibara et al., 2002). Both MSIs are expressed in the developing and adult nervous system. In mammals, MSI1 is mainly expressed in stem and progenitor cells and its expression decreases during differentiation (Sakakibara et al., 2001), whereas MSI2 is present also in differentiated neurons of the adult brain (Sakakibara et al., 2001). In nematodes, the sole musashi (msi-1) is widely expressed during embryogenesis and remains present in differentiated mature neurons of the adult nervous system similar to musashi inDrosophila (Hirota et al., 1999, Yoda et al., 2000). In C. elegans, loss of the msi-1gene causes a defect in male mating behavior (Yoda et al., 2000), suggesting that MSI may regulate the activity of differentiated neurons.
MSIs bind to the (G/A)UnAGU (n = 1–3) motif located in the 3′ UTR of the target mRNA. Although MSI binding to this RNA sequence in vitro is well documented (Ohyama et al., 2012), so far only few in vivo targets were identified, such as m-numb (Imai et al., 2001), CDKN1A (Battelli et al., 2006), doublecortin (Horisawa et al., 2009), and c-mos in Xenopus leavis (Charlesworth et al., 2006). Beside these, an immunoprecipitation of RNA-binding protein coupled to microarray (RIP-ChIP) approach recently identified 64 mRNAs that were interacting with MSI in transfected human embryonic kidney 293 cells (de Sousa Abreu et al., 2009). These MSI-binding partners are mainly genes involved in proliferation, apoptosis, cell differentiation, and posttranslational modification and, interestingly, include a component of the Arp2/3 actin branching regulator protein complex (ACTR2). Thus, its expression pattern in the nervous system and its interaction with theACTR2/arx-2 mRNA make Musashi a likely candidate that may regulate memory.
Here, we show that the C. elegans neuronal musashi gene ortholog msi-1regulates forgetting. Although MSI-1 is expressed in several neurons, memory length depends on the action of MSI-1 only in the AVA interneuron. We demonstrate that MSI-1 binds in vivo to the mRNA of three members of the actin branching ARP2/3 complex and regulates their protein levels via a 3′ UTR-dependent translational repression. The inhibitory function of msi-1 is also reflected in persistence of GLR-1-positive synapse size increase induced by associative learning in msi-1(lf) mutants. Finally, GLR-1 signaling possibly regulates both actin capping through the activity of adducin (add-1) and inhibition of actin branching mediated by msi-1, and these two parallel mechanisms act in concert to establish the proper memory trace. Our results suggest that MSI-1 regulates forgetting and point to a novel aspect of memory regulation linking translational repression to regulation of the actin cytoskeleton structure.
Hadziselimovic, N., Vukojevic, V., Peter, F., Milnik, A., Fastenrath, M., Fenyves, B., Hieber, P., Demougin, P., Vogler, C., de Quervain, D., Papassotiropoulos, A. and Stetak, A. (2014). Forgetting Is Regulated via Musashi-Mediated Translational Control of the Arp2/3 Complex. Cell, 156(6), pp.1153-1166.
Neurons in human skin perform advanced calculations similar to those in the brain (cerebral cortex)
Edge-orientation processing in first-order tactile neurons
A fundamental feature of first-order neurons in the tactile system is that their distal axon branches in the skin and forms many transduction sites, yielding complex receptive fields with many highly sensitive zones. We found that this arrangement constitutes a peripheral neural mechanism that allows individual neurons to signal geometric features of touched objects. Specifically, we observed that two types of first-order tactile neurons that densely innervate the glabrous skin of the human fingertips signaled edge orientation via both the intensity and the temporal structure of their responses. Moreover, we found that the spatial layout of a neuron’s highly sensitive zones predicted its sensitivity to particular edge orientations. We submit that peripheral neurons in the touch-processing pathway, as with peripheral neurons in the visual-processing pathway, perform feature extraction computations that are typically attributed to neurons in the cerebral cortex.
Pruszynski, J. and Johansson, R. (2014). Edge-orientation processing in first-order tactile neurons. Nature Neuroscience, 17(10), pp.1404-1409.
Study provides new insight into what occurs in the brain during the learning process
With the neurological correlates of the learning process coming into focus, the scientists were able to delve into the differences among participants in order to explain why some learned the sequences faster than others. Counterintuitive as it may seem, the participants who showed decreased neural activity learned the fastest. The critical distinction was in areas not directly related to seeing the cues or playing the notes: the frontal cortex and the anterior cingulate cortex.
These cognitive control centers are thought to be most responsible for what is known as executive function. “This neurological trait is associated with making and following through with plans, spotting and avoiding errors and other higher-order types of thinking,” Grafton said. “In fact, good executive function is necessary for complex tasks but might actually be a hindrance to mastering simple ones.”
Grafton also noted that the frontal cortex and the anterior cingulate cortex are among the last brain regions to fully develop in humans, which may help explain why children are able to acquire new skills quickly as compared to adults.
“It’s the people who can turn off the communication to these parts of their brain the quickest who have the steepest drop-off in their completion times,” said Bassett. “It seems like those other parts are getting in the way for the slower learners. It’s almost like they’re trying too hard and overthinking it.”
Additional studies will delve into why some people are better than others at shutting down the connections in these parts of the brains.
Bassett, D., Yang, M., Wymbs, N. and Grafton, S. (2015). Learning-induced autonomy of sensorimotor systems. Nature Neuroscience, 18(5), pp.744-751.
___________________________________________________
Neural Basis of Sensorimotor Plasticity in Speech Motor Adaptation
Abstract:
When we speak, we get correlated sensory feedback from speech sounds and from the muscles and soft tissues of the vocal tract. Here we dissociate the contributions of auditory and somatosensory feedback to identify brain networks that underlie the somatic contribution to speech motor learning. The technique uses a robotic device that selectively alters somatosensory inputs in combination with resting-state fMRI scans that reveal learning-related changes in functional connectivity. A partial correlation analysis is used to identify connectivity changes that are not explained by the time course of activity in any other learning-related areas. This analysis revealed changes related to behavioral improvements in movement and separately, to changes in auditory perception: Speech motor adaptation itself was associated with connectivity changes that were primarily in non-motor areas of brain, specifically, to a strengthening of connectivity between auditory and somatosensory cortex and between presupplementary motor area and the inferior parietal lobule. In contrast, connectively changes associated with alterations to auditory perception were restricted to speech motor areas, specifically, primary motor cortex and inferior frontal gyrus. Overall, our findings show that during adaptation, somatosensory inputs result in a broad range of changes in connectivity in areas associated with speech motor control and learning.
Darainy, M., Vahdat, S. and Ostry, D. (2018). Neural Basis of Sensorimotor Plasticity in Speech Motor Adaptation. Cerebral Cortex, 29(7), pp.2876-2889.
What Bat Brains Might Tell Us About Human Brains
Could a new finding in bats help unlock a mystery about the human brain? Likely so, say researchers at Georgetown University Medical Center who have shown that a small region within the amygdala, an almond-shaped structure in the brains of all mammals, is responsible for producing emotional calls and sounds. They say this discovery might be key to locating a similar center in human brains.
Localizing and manipulating this center in the human brain may provide a way to treat malfunctions in emotional responses, resulting, for example, in pathological aggression, says the study’s senior investigator, Jagmeet S. Kanwal, PhD, associate professor of neurology at Georgetown. On the flip side, he says it may be possible to “give voice to the voiceless — allow those who are deeply withdrawn, perhaps even mute due to aberrant wiring in the amygdala, to speak.”
Research points to the possibility of such a center in humans: functional imaging has already shown that the human amygdala —like the bat’s amygdala— responds to species-specific emotive sounds, such as laughing and crying, Kanwal says.
Article: What bat brains might tell us about human brains
Easily distracted by noise? You might be a creative genius
The literary great Marcel Proust wore ear-stoppers because he was unable to filter out irrelevant noise — and lined his bedroom with cork to attenuate sound.
Now new Northwestern University research suggests why the inability to shut out competing sensory information while focusing on the creative project at hand might have been so acute for geniuses such as Proust, Franz Kafka, Charles Darwin, Anton Chekhov and many others.
The Northwestern research provides the first physiological evidence that real-world creativity may be associated with a reduced ability to filter “irrelevant” sensory information.
The research suggests that some people are more affected by the daily bombardment of sensory information — or have “leakier” sensory filters.
Creativity and sensory gating indexed by the P50: Selective versus leaky sensory gating in divergent thinkers and creative achievers
Creativity has previously been linked with atypical attention, but it is not clear what aspects of attention, or what types of creativity are associated. Here we investigated specific neural markers of a very early form of attention, namely sensory gating, indexed by the P50 ERP, and how it relates to two measures of creativity: divergent thinking and real-world creative achievement.
Data from 84 participants revealed that divergent thinking (assessed with the Torrance Test of Creative Thinking) was associated with selective sensory gating, whereas real-world creative achievement was associated with “leaky” sensory gating, both in zero-order correlations and when controlling for academic test scores in a regression. Thus both creativity measures related to sensory gating, but in opposite directions.
Additionally, divergent thinking and real-world creative achievement did not interact in predicting P50 sensory gating, suggesting that these two creativity measures orthogonally relate to P50 sensory gating. Finally, the ERP effect was specific to the P50 – neither divergent thinking nor creative achievement were related to later components, such as the N100 and P200.
Overall results suggest that leaky sensory gating may help people integrate ideas that are outside of focus of attention, leading to creativity in the real world; whereas divergent thinking, measured by divergent thinking tests which emphasize numerous responses within a limited time, may require selective sensory processing more than previously thought.
Zabelina, D., O’Leary, D., Pornpattananangkul, N., Nusslock, R. and Beeman, M. (2015). Creativity and sensory gating indexed by the P50: Selective versus leaky sensory gating in divergent thinkers and creative achievers. Neuropsychologia, 69, pp.77-84.
___________________________________________
“There is no great genius without some touch of madness.” -Seneca
Creativity and psychosis share genetic roots
We tested whether polygenic risk scores for schizophrenia and bipolar disorder would predict creativity. Higher scores were associated with artistic society membership or creative profession in both Icelandic (P = 5.2 × 10−6 and 3.8 × 10−6 for schizophrenia and bipolar disorder scores, respectively) and replication cohorts (P = 0.0021 and 0.00086). This could not be accounted for by increased relatedness between creative individuals and those with psychoses, indicating that creativity and psychosis share genetic roots.
Power, R., Steinberg, S., Bjornsdottir, G., Rietveld, C., Abdellaoui, A., Nivard, M., Johannesson, M., Galesloot, T., Hottenga, J., Willemsen, G., Cesarini, D., Benjamin, D., Magnusson, P., Ullén, F., Tiemeier, H., Hofman, A., van Rooij, F., Walters, G., Sigurdsson, E., Thorgeirsson, T., Ingason, A., Helgason, A., Kong, A., Kiemeney, L., Koellinger, P., Boomsma, D., Gudbjartsson, D., Stefansson, H. and Stefansson, K. (2015). Polygenic risk scores for schizophrenia and bipolar disorder predict creativity. Nature Neuroscience, 18(7), pp.953-955.
___________________________________________
___________________________________________
Neural correlates of creative insight: Amplitude of low-frequency fluctuation of resting-state brain activity predicts creative insight
Abstract:
Creative insight has attracted much attention across cultures. Although previous studies have explored the neural correlates of creative insight by functional magnetic resonance imaging (fMRI), little is known about intrinsic resting-state brain activity associated with creative insight. In the present study, we used amplitude of low-frequency fluctuation (ALFF) as an index in resting-state fMRI (rs-fMRI) to identify brain regions involved in individual differences in creative insight, which was measured by the response time of creative Chinese character chunk decomposition. Our results showed that ALFF in the superior frontal gyrus (SFG) positively predicted creative insight, while ALFF in the middle cingulate cortex/insula cortex (MCC/IC), superior temporal gyrus/angular gyrus (STG/AG), anterior cingulate cortex/caudate nucleus (ACC/CN), and culmen/declive (CU/DC) negatively predicted creative insight. Moreover, these findings indicate that spontaneous brain activity in multiple regions related to breaking mental sets, solutions exploring, evaluation of novel solutions, forming task-related associations, and emotion experience contributes to creative insight. In conclusion, the present study provides new evidence to further understand the cognitive processing and neural correlates of creative insight.
Lin, J., Cui, X., Dai, X., Chen, Y. and Mo, L. (2019). Neural correlates of creative insight: Amplitude of low-frequency fluctuation of resting-state brain activity predicts creative insight. PLOS ONE, 14(2), p.e0213212.
___________________________________________
Robust prediction of individual creative ability from brain functional connectivity
Significance:
People’s capacity to generate creative ideas is central to technological and cultural progress. Despite advances in the neuroscience of creativity, the field lacks clarity on whether a specific neural architecture distinguishes the highly creative brain. Using methods in network neuroscience, we modeled individual creative thinking ability as a function of variation in whole-brain functional connectivity. We identified a brain network associated with creative ability comprised of regions within default, salience, and executive systems—neural circuits that often work in opposition. Across four independent datasets, we show that a person’s capacity to generate original ideas can be reliably predicted from the strength of functional connectivity within this network, indicating that creative thinking ability is characterized by a distinct brain connectivity profile.
Abstract:
People’s ability to think creatively is a primary means of technological and cultural progress, yet the neural architecture of the highly creative brain remains largely undefined. Here, we employed a recently developed method in functional brain imaging analysis—connectome-based predictive modeling—to identify a brain network associated with high-creative ability, using functional magnetic resonance imaging (fMRI) data acquired from 163 participants engaged in a classic divergent thinking task. At the behavioral level, we found a strong correlation between creative thinking ability and self-reported creative behavior and accomplishment in the arts and sciences (r = 0.54). At the neural level, we found a pattern of functional brain connectivity related to high-creative thinking ability consisting of frontal and parietal regions within default, salience, and executive brain systems. In a leave-one-out cross-validation analysis, we show that this neural model can reliably predict the creative quality of ideas generated by novel participants within the sample. Furthermore, in a series of external validation analyses using data from two independent task fMRI samples and a large task-free resting-state fMRI sample, we demonstrate robust prediction of individual creative thinking ability from the same pattern of brain connectivity. The findings thus reveal a whole-brain network associated with high-creative ability comprised of cortical hubs within default, salience, and executive systems—intrinsic functional networks that tend to work in opposition—suggesting that highly creative people are characterized by the ability to simultaneously engage these large-scale brain networks.
Beaty, R., Kenett, Y., Christensen, A., Rosenberg, M., Benedek, M., Chen, Q., Fink, A., Qiu, J., Kwapil, T., Kane, M. and Silvia, P. (2018). Robust prediction of individual creative ability from brain functional connectivity. Proceedings of the National Academy of Sciences, 115(5), pp.1087-1092.
Danielle Bassett: The Flexible Brain
As an undergraduate, Danielle Bassett was driven by a passion for math and physics. But when a close friend attempted suicide, Bassett recognized that she could use her knowledge of physics and math to further what scientists understand about the brain.
Article: http://www.brainfacts.org/about-neuroscience/meet-the-researcher/articles/2014/danielle-bassett-the-flexible-brain/
Brain activity boosts processes that promote neural connections
Neuronal activity biases axon selection for myelination in vivo
An essential feature of vertebrate neural development is ensheathment of axons with myelin, an insulating membrane formed by oligodendrocytes. Not all axons are myelinated, but mechanisms directing myelination of specific axons are unknown. Using zebrafish, we found that activity-dependent secretion stabilized myelin sheath formation on select axons. When VAMP2-dependent exocytosis was silenced in single axons, oligodendrocytes preferentially ensheathed neighboring axons.
Nascent sheaths formed on silenced axons were shorter in length, but when activity of neighboring axons was also suppressed, inhibition of sheath growth was relieved.
Using in vivotime-lapse microscopy, we found that only 25% of oligodendrocyte processes that initiated axon wrapping were stabilized during normal development and that initiation did not require activity. Instead, oligodendrocyte processes wrapping silenced axons retracted more frequently.
We propose that axon selection for myelination results from excessive and indiscriminate initiation of wrapping followed by refinement that is biased by activity-dependent secretion from axons.
Hines, J., Ravanelli, A., Schwindt, R., Scott, E. and Appel, B. (2015). Neuronal activity biases axon selection for myelination in vivo. Nature Neuroscience, 18(5), pp.683-689.
____________________________________________
Sweet taste liking is associated with impulsive behaviors in humans
Evidence from both human and animal studies suggests that sensitivity to rewarding stimuli is positively associated with impulsive behaviors, including both impulsive decision making and inhibitory control.
The current study examined associations between the hedonic value of a sweet taste and two forms of impulsivity (impulsive choice and impulsive action) in healthy young adults (N = 100). Participants completed a sweet taste test in which they rated their liking of various sweetness concentrations. Subjects also completed measures of impulsive choice (delay discounting), and impulsive action (go/no-go task).
Subjects who discounted more steeply (i.e., greater impulsive choice) liked the high sweetness concentration solutions more. By contrast, sweet liking was not related to impulsive action. These findings indicate that impulsive choice may be associated with heightened sensitivity to the hedonic value of a rewarding stimulus, and that these constructs might share common underlying neurobiological mechanisms.
Weafer, J., Burkhardt, A. and de Wit, H. (2014). Sweet taste liking is associated with impulsive behaviors in humans. Front. Behav. Neurosci., 8.
____________________________________________
Being in love makes water tastes sweeter
Scientific proof that love is a “sweet thing”.
Thinking about love made the plain, distilled water seem sweeter than thinking about jealousy or happiness.
Why? The researchers hypothesize that it could have to do with the shared neural reward circuitry associated with experiencing both love and sweetness.
Studies have found the anterior cingulate cortex, a brain region which plays a role in anticipating reward, is activated by looking at pictures of romantic partners and by tasting sugar. “Therefore, it is possible that when one experiences love, the anterior cingulate cortex would activate representations associated with sweetness, thereby eliciting sweetness sensations even without actual sweetness input from an external source,” the researchers write.
Article: http://www.popsci.com/article/science/being-love-makes-water-taste-sweeter
____________________________________________
Oxytocin may be key to lasting relationships
Oxytocin enhances brain reward system responses in men viewing the face of their female partner
Sexual monogamy is potentially costly for males, and few mammalian species along with humans exhibit it. The hypothalamic peptide oxytocin (OXT) has been implicated in mediating pair bonds in various species, but as yet, we know little about neurobiological factors that might act to promote fidelity, especially in men. Here we provide evidence for a mechanism by which OXT may contribute to romantic bonds in men by enhancing their partner’s attractiveness and reward value compared with other women.
The biological mechanisms underlying long-term partner bonds in humans are unclear. The evolutionarily conserved neuropeptide oxytocin (OXT) is associated with the formation of partner bonds in some species via interactions with brain dopamine reward systems. However, whether it plays a similar role in humans has as yet not been established.
Here, we report the results of a discovery and a replication study, each involving a double-blind, placebo-controlled, within-subject, pharmaco-functional MRI experiment with 20 heterosexual pair-bonded male volunteers. In both experiments, intranasal OXT treatment (24 IU) made subjects perceive their female partner’s face as more attractive compared with unfamiliar women but had no effect on the attractiveness of other familiar women. This enhanced positive partner bias was paralleled by an increased response to partner stimuli compared with unfamiliar women in brain reward regions including the ventral tegmental area and the nucleus accumbens (NAcc). In the left NAcc, OXT even augmented the neural response to the partner compared with a familiar woman, indicating that this finding is partner-bond specific rather than due to familiarity.
Taken together, our results suggest that OXT could contribute to romantic bonds in men by enhancing their partner’s attractiveness and reward value compared with other women…
Scheele, D., Wille, A., Kendrick, K., Stoffel-Wagner, B., Becker, B., Gunturkun, O., Maier, W. and Hurlemann, R. (2013). Oxytocin enhances brain reward system responses in men viewing the face of their female partner. Proceedings of the National Academy of Sciences, 110(50), pp.20308-20313.
Spotlight on science: Study reveals the brain’s white matter “scaffold” of connections
Neuroscientists have introduced new terminology to describe the brain’s connective neural pathways after completing a study that used social network models to analyze the brain’s communication network. Researchers at USC Institute for Neurology and Informatics are now calling the brain’s network of white matter a “scaffold” of connections through which the brain operates.
Article: http://blog.cambridgesoft.com/post/2014/02/21/Spotlight-on-science-Study-reveals-the-brains-white-matter-scaffold-of-connections-.aspx
Mindfulness practice leads to increases in regional brain gray matter density
Therapeutic interventions that incorporate training in mindfulness meditation have become increasingly popular, but to date, little is known about neural mechanisms associated with these interventions. Mindfulness-Based Stress Reduction (MBSR), one of the most widely used mindfulness training programs, has been reported to produce positive effects on psychological well-being and to ameliorate symptoms of a number of disorders. Here, we report a controlled longitudinal study to investigate pre-post changes in brain gray matter concentration attributable to participation in an MBSR program. Anatomical MRI images from sixteen healthy, meditation-naïve participants were obtained before and after they underwent the eight-week program.
Changes in gray matter concentration were investigated using voxel-based morphometry, and compared to a wait-list control group of 17 individuals. Analyses in a priori regions of interest confirmed increases in gray matter concentration within the left hippocampus. Whole brain analyses identified increases in the posterior cingulate cortex, the temporo-parietal junction, and the cerebellum in the MBSR group compared to the controls.
The results suggest that participation in MBSR is associated with changes in gray matter concentration in brain regions involved in learning and memory processes, emotion regulation, self-referential processing, and perspective taking.
Hölzel, B., Carmody, J., Vangel, M., Congleton, C., Yerramsetti, S., Gard, T. and Lazar, S. (2011). Mindfulness practice leads to increases in regional brain gray matter density. Psychiatry Research: Neuroimaging, 191(1), pp.36-43.
____________________________________
How Does Meditation Change the Brain?
____________________________________
Hippocampal volume in vulnerability and resilience to depression
Our overall results suggest that reduced hippocampal volumes is a neural marker for the scar effect of depression, although this structural impairment could also be seen as a vulnerability marker for the development of future recurrent episodes. By contrast, larger hippocampal volumes could be a biological marker of resilience. These findings have clinical implications regarding treatment development for the prevention of illness onset and recurrent depressive episodes.
Chan, S., Harmer, C., Norbury, R., O’Sullivan, U., Goodwin, G. and Portella, M. (2016). Hippocampal volume in vulnerability and resilience to depression. Journal of Affective Disorders, 189, pp.199-202.
Cognitive performance during long-term residence in a polar environment
Extract:
The objective of this study was to determine whether there was significant change in cognitive performance by prolonged duration of residence in polar environment. We examined volunteers (N = 23, scientific and logistic personnel) who spent fourteen months of continuous stay at ‘Maitri’ – The Indian research base in Antarctica (70 °45′S, 11 °44′E). Cognitive measures (tests of task acquisition, delayed recognition, attention and concentration, and digit symbol substitution) were obtained at the beginning (second month), the middle (seventh month) and the final (twelfth month) phases of prolonged residence in Antarctica.
A repeated-measures MANOVA was conducted and the findings indicated a significant increase in percent accuracy in performance of task acquisition, delayed recognition and digit symbol tests that assessed recognition memory and learning. The test of attention and concentration that measured short-term memory for digits showed stable performance over a long-duration of residence in polar environment.
Findings indicated positive as well as neutral effect on cognitive performance of winter-over residents. The tasks measuring recognition memory and learning showed an increased accuracy over a long-duration. This finding is consistent with some of the previous reports that observed improvement in cognitive performance (for example, Defayolle et al., 1985, Suedfeld et al., 1987, Palinkas et al., 2005 and Mäkinen et al., 2006). However, the task measuring short-term memory for digit showed neither reduction nor improvement from the beginning to the final phases of long-duration of residence.
This result is in line with the work by Suedfeld (1969) who upon reviewing some consistent findings concluded that no significant decrement occurs on digit span as a result of any of sensory deprivation. Further, quite a few studies reported similar finding that cognitive performance did not reduce over time in isolated and extreme environment (for instance, Brady and Emurian, 1978, Suedfeld, 1980 and Marrao et al., 2005).
John Paul, F., Mandal, M., Ramachandran, K. and Panwar, M. (2010). Cognitive performance during long-term residence in a polar environment. Journal of Environmental Psychology, 30(1), pp.129-132.
https://twitter.com/NeuroscienceNew/status/895707364061462528
“Ce sont ceux qui savent peu, et pas ceux qui en savent beaucoup, qui affirment si positivement que tel ou tel problème ne sera jamais résolu par la science.” -Charles Darwin
____________________________________________
____________________________________________
Sequence Alterations of Cortical Genes Linked to Individual Connectivity of the Human Brain
Abstract:
Individual differences in humans are driven by unique brain structural and functional profiles, presumably mediated in part through differential cortical gene expression. However, the relationships between cortical gene expression profiles and individual differences in large-scale neural network organization remain poorly understood. In this study, we aimed to investigate whether the magnitude of sequence alterations in regional cortical genes mapped onto brain areas with high degree of functional connectivity variability across individuals. First, human genetic expression data from the Allen Brain Atlas was used to identify protein-coding genes associated with cortical areas, which delineated the regional genetic signature of specific cortical areas based on sequence alteration profiles. Thereafter, we identified brain regions that manifested high degrees of individual variability by using test-retest functional connectivity magnetic resonance imaging and graph-theory analyses in healthy subjects. We found that rates of genetic sequence alterations shared a distinct spatial topography with cortical regions exhibiting individualized (highly-variable) connectivity profiles. Interestingly, gene expression profiles of brain regions with highly individualized connectivity patterns and elevated number of sequence alterations are devoted to neuropeptide-signaling-pathways and chemical-synaptic-transmission. Our findings support that genetic sequence alterations may underlie important aspects of brain connectome individualities in humans.
Significance Statement: The neurobiological underpinnings of our individuality as humans are still an unsolved question. Although the notion that genetic variation drives an individual’s brain organization has been previously postulated, specific links between neural connectivity and gene expression profiles have remained elusive. In this study, we identified the magnitude of population-based sequence alterations in discrete cortical regions and compared them to the brain topological distribution of functional connectivity variability across an independent human sample. We discovered that brain regions with high degree of connectional individuality are defined by increased rates of genetic sequence alterations; these findings specifically implicated genes involved in neuropeptide-signaling pathways and chemical-synaptic transmission. These observations support that genetic sequence alterations may underlie important aspects of the emergence of the brain individuality across humans.
Xin, Q., Ortiz-Terán, L., Diez, I., Perez, D., Ginsburg, J., El Fakhri, G. and Sepulcre, J. (2018). Sequence Alterations of Cortical Genes Linked to Individual Connectivity of the Human Brain. Cerebral Cortex, 29(9), pp.3828-3835.
____________________________________________
Scientists discover 1,000 new “intelligence genes”
Intelligence is associated with important economic and health-related life outcomes1. Despite intelligence having substantial heritability2 (0.54) and a confirmed polygenic nature, initial genetic studies were mostly underpowered3,4,5. Here we report a meta-analysis for intelligence of 78,308 individuals. We identify 336 associated SNPs (METAL P < 5 × 10−8) in 18 genomic loci, of which 15 are new. Around half of the SNPs are located inside a gene, implicating 22 genes, of which 11 are new findings. Gene-based analyses identified an additional 30 genes (MAGMA P < 2.73 × 10−6), of which all but one had not been implicated previously. We show that the identified genes are predominantly expressed in brain tissue, and pathway analysis indicates the involvement of genes regulating cell development (MAGMA competitive P = 3.5 × 10−6). Despite the well-known difference in twin-based heritability2 for intelligence in childhood (0.45) and adulthood (0.80), we show substantial genetic correlation (rg = 0.89, LD score regression P = 5.4 × 10−29). These findings provide new insight into the genetic architecture of intelligence.
Sniekers, S., Stringer, S., Watanabe, K., Jansen, P., Coleman, J., Krapohl, E., Taskesen, E., Hammerschlag, A., Okbay, A., Zabaneh, D., Amin, N., Breen, G., Cesarini, D., Chabris, C., Iacono, W., Ikram, M., Johannesson, M., Koellinger, P., Lee, J., Magnusson, P., McGue, M., Miller, M., Ollier, W., Payton, A., Pendleton, N., Plomin, R., Rietveld, C., Tiemeier, H., van Duijn, C. and Posthuma, D. (2017). Genome-wide association meta-analysis of 78,308 individuals identifies new loci and genes influencing human intelligence. Nature Genetics, 49(7), pp.1107-1112.
____________________________________________
Clinical Psychology: Learning Disabilities, Anxiety, Depression & Schizophrenia and the Effectiveness of Psychotherapy
CAMHS deal with the psychological issues of people under the age of 18. They are a non-specialist service and often refer to other more specialised departments following the initial assessment of patients. The most common cases tend to be adolescents with depression and anxiety whose manifestations are not different to those of adults and so are treated fairly similarly.
Inclusivism in Learning Disabilities
In 1969, Bengt Nirje adopted and developed the concept of normalisation in Sweden and beautifully described it as…
“making available to all mentally retarded people patterns of life and conditions of everyday living which are as close as possible to the regular circumstances and ways of life of society.”
– Nirje, 1980
Learning Disability is not just an impairment in Cognition
The social impairment of Learning Disabilities – US Statute 111 – 256: Rosa’s Law defines the factual impairment, the imposed or acquired disability and the awareness of being different.
The Normalisation Theory
This theory focuses on the mainstream social trends of social devaluation or deviancy making. Some categories of people tend to be valued negatively due to their behaviours, appearances and characteristics, and this places them at the risk of being devalued [according to the Normalisation Theory of Nirje on the societal processes he assumed] – people fulfil various social roles and stereotypes. As part of the deviancy making or social devaluation, the unsophisticated minds of the masses generally do not mean to stereotype, however they seem to do it unconsciously [the unconscious is a concept Sigmund Freud and Jacques Lacan acknowledged in their psychoanalytic theories of mental/psychological activity and its disorders land mental health problems linked to psychopathic tendencies in people towards others], i.e. deviant groups with social symbols or images that are at a higher risk of being devalued are the focus of the normalisation theory, which is believed to be done with the aim of providing them with the skills they need and eventually change the status of these deviant groups.
Society tends to distance itself from deviant groups without any purpose or belonging, however psychologists provide support for the social integration and valued social participation of people with learning disabilities through exercises that involve learning through imitation. This challenges stereotypes within wider society through direct experiences of spending time with people who are affected by learning disabilities.
While psychology evolves and sophisticated and modern theories about intelligence and communication such as our “Organic Theory” take shape, we hope that observations such as this one may be digested and understood by the masses, that is:
“While the communicative patterns [language] in human primates vary with socio-behavioural and geographical patterns; creativity and IQ remain constant and do not change. Intelligence and creativity cannot be stopped because of linguistic differences, since talented and gifted humans do not choose the location of their birth nor their linguistic heritage but still contribute to the enhancement of our civilisation.”
Which concludes that that the intelligence of an invidual when assessed on a range of variables [e.g. perception, fluid intelligence, reasoning, emotional intelligence, courage, etc] cannot be deduced by simply assessing their academic abilities, since human life has various sides to itself. Hence, the true worth and value of an individual may always remain a problem and a mystery to fully assess, and this seems to go in line with Jean Piaget’s deduction about the uniqueness of the human organism and mind.
Full Article: https://dpurb.com/2018/07/02/clinical-psychology-learning-disabilities-anxiety-depression-schizophrenia-and-the-effectiveness-of-psychotherapy/